CRISPR/CAS9 technology in the field of cell therapy
一、Introduction of CRISPR/Cas system
CRISPR (Clustered Regularly Interspaced Short Palindromic Repeat), first discovered in the late 1980s, is an unusual genetic structure made up of alternating and non-repeating DNA sequences. The CRISPR/Cas system is essentially a bacterial defense mechanism against foreign DNA. Genomic analysis has shown that CRISPR and Cas proteins function as an acquired immune system and protect prokaryotic DNA from attack by phage and plasmid DNA through an RNA-guided DNA cutting system [1, 2].
CRISPR/Cas system has a variety of categories, of which CRISPR/Cas9 system is the most in-depth research, the most mature technology, the most widely used category. The CRISPR/Cas9 system consists of a single-stranded guide RNA (sgRNA) and a Cas9 protein that functions as an endonucrenase. Under the specific recognition of sgRNA, Cas9 protein reached a specific site of the genome and cut double-stranded DNA (dsDNA), resulting in double-strand break (DSB). DSBS are then repaired by cell-independent nonhomologous DNA end joining (NHEJ) or homology-directed repair (HDR). Among them, the dominant NHEJ repair is prone to mutation [3].
Figure 1. How the CRISPR/Cas9 system works (image from biorender)
二、CRISPR/Cas9 system delivery technology
The natural Cas protein still has many limitations in terms of editing efficiency and specificity, which may cut DNA double strands at misplaced loci, resulting in potential risks that significantly affect its practical application. Researchers have developed different Cas9 variants to reduce the mismatch rate and increase editing efficiency [4-6].
The delivery method of the CRISPR/Cas9 system is also one of the key factors affecting the efficiency of gene editing [7]. There are three main forms of delivery: (1) delivery of plasmid DNA encoding Cas9 and sgRNA; Delivery of Cas9 mRNA and sgRNA components; Delivery of RNP(ribonucleoprotein,Cas9 protein and sgRNA complex). The selection of different delivery modes according to different delivery forms can be divided into three main groups according to the cell entry mechanism: (1) biological methods, (2) chemical methods, and (3) physical methods. Biological methods use natural biological materials such as viral proteins, peptides or cell receptors/membranes to mediate entry into cells. This category includes viral vectors, virus-like particles (VLPs), and cell-penetrating peptides (CPPs). Chemical methods use synthetic materials, such as polymers, lipids, or metals, to facilitate entry into cells. This category includes liposomes, gold nanoparticles (AuNPs), and lipid nanoparticles (LNPs). Physical methods rely on the physical energy of electricity or ultrasound to deliver genes into cells. This category includes electroporation, sound hole effects, and microinjection.
三、CRISPR/Cas9 technology in gene and cell therapy research progress
Compared with the first and second generation gene editing technologies, CRISPR/Cas9 technology has been widely used in biomedical research due to its excellent performance in gene knockout, gene silencing, gene knockout, gene activation and epigenetic modification due to its convenient, simple and efficient operation. A report by Transparency Market Research (TMR) entitled "CRISPR and Cas Gene Market - Global Industry Analysis, Size, Share, Growth, Trends and Forecasts, 2018-2026" says that by 2026, The global CRISPR and Cas gene market is valued at $7.234.5 billion, with a CAGR of approximately 20.1% from 2018 to 2026. The use of gene editing technology for the prevention of multiple diseases, along with the increasing demand for alternative medicine for chronic diseases and increased investment by key players in Asia Pacific are expected to be the major factors driving the market from 2018 to 2026.
(1) CRISPR/Cas9 genome-wide functional screening
By constructing CRISPR-Cas9 library, some drug targets and resistance genes can be screened, analyzed and verified on a large scale, and relevant data can be provided for disease treatment. The researchers used CRISPR-Cas9 library to screen the whole genome of human gastric cancer AGS cells for potential therapeutic targets, and finally identified 41 essential genes as potential drug targets [8]. In addition, CRISPR-Cas9 library was used to screen 685 gene loci of the key transcription factor p53 and the enhancer element of ERα in cancer cells, and a total of 3 enhancer elements were identified to be related to ERα. Three enhancer elements are associated with p53 function, and only when two enhancer elements are fully combined with p53 can the cell aging process be activated [9].
(2) Model construction
Disease models are mainly used to simulate the biological and pathological characteristics of human diseases, and play a key role in the pathogenesis of diseases and drug screening.
Most animal models are constructed by knocking out specific genes [10]. Using CRISPR technology to target exon 3 of the monkey SHANK21 gene, researchers successfully constructed an ASD model of the monkey and its F1 offspring due to SHANK3 gene mutation, showing atypical autism phenotypes such as increased repetitive behaviors and social and learning deficits [11]. Point mutation knockin based on single base editing has great potential in building animal models. The researchers ended gene expression early by making single-base edits to stop codons at RAG1, RAG2, and IL2RG sites. After these embryos were implanted into surrogate pigs, it was found that piglets carrying homozygous or heterozygous mutations would suffer from severe underdevelopment of the thymus and spleen, resulting in immune deficiency and lung infection. It was also the first time that single-base editing techniques had been used in large animals [12]. In addition, the construction of cell models in vitro also helps to screen drug action targets and provide basis for revealing drug action mechanisms and disease mechanisms.
(3) Cell therapy
Immunotherapy is a method of killing tumor cells by mobilizing the patient's own immune system, which is mainly divided into immune checkpoint blocking and chimeric antigen receptor T-cell immunotherapy (CAR-T). CRISPR/Cas9 technology is different from the random integration of transgenic technology, which can accurately target target genes and carry out genetic modification. Due to the presence of endogenous HLA and TCR in allogeneic CAR-T cells, which hinder the wide application of therapy, multiple therapeutic strategies have been proposed to prepare CAR-T. Consider the important role of granulocyte-macrophage colony-stimulating factor (GM-CSF) in inducing cytokine storms. In 2019, Sterner et al. [13] used CRISPR/Cas9 to knock out the GM-CSF gene of CD19 CAR-T cells. The results of this study showed that GM-CSF secretion of CAR T cells with GM-CSF gene knockout was indeed significantly reduced, but the important function of CAR T cells was not affected. More importantly, GM-CSF-deficient CD19 CAR T cells showed significant anti-tumor effects in vivo compared with wild-type CD19 CAR T cells. In 2020, Carl June et al. [14] removed endogenous T cell receptor (TCR) and immune checkpoint molecule programmed cell death protein 1 (PD-1) to improve the function and persistence of engineered T cells. A synthetic cancer-specific TCR transgene (NY-ESO-1) was introduced to identify tumor cells (the first multiple CRISPR/Cas9 editing engineering applied to clinical trials). In the same year, the results of the first human phase I clinical trial of CRISPR-Cas9-edited PD-1 T cells in patients with advanced non-small cell lung cancer (ClinicalTrials.gov NCT02793856) were obtained by Professor Lu Youu of China, and the remaining clinical trials were shown in the following table [15].
(4) Gene therapy
Gene therapy refers to the introduction of foreign normal genes into target cells to correct or compensate for diseases caused by defects and abnormal genes in order to achieve therapeutic purposes. Thanks to its high editing efficiency and the ability to simultaneously target multiple target genes, CRISPR/Cas9 technology has been used in gene therapy for diseases such as tumors, neurodegenerative diseases, hematopoietic diseases, and AIDS.
CRISPR Therapeutics has announced the early success of its treatment, called CTX001, which delivers CRISPR-Cas9 to blood stem cells via electroporation. After the patient's own hematopoietic stem cells were chemically cleared with leucoamine, the CRISPR-Cas9-edited hematopoietic stem cells were transfused back into the patient. Patients with beta-thalassemia or sickle cell disease who are treated are no longer dependent on blood transfusion therapy for a long time [16].
NTLA-2001 is being developed as a single-dose treatment for transthyroxin protein (ATTR) amyloidosis. NTLA-2001 is an in vivo gene-editing therapy designed to treat ATTR amyloidosis by reducing the concentration of TTR in the serum. The therapy is based on CRISPR/Cas, which is the first CRISPR therapy to be delivered into the bloodstream in the form of lipid nanoparticles for systemic administration [17].
Editas Medicine is developing EDIT-101, a product that is still in clinical trials. The drug uses AAV-mediated delivery of CRISPR/Cas9, targeted by sgRNA to remove the abnormal splicing donor resulting from the IVS290 mutation in the CEP26 gene, and restores normal CEP290 expression to treat congenital amaurosis. The treatment can be cured with a single dose (NCT03872479).
Reference:
[1] RAN F A, HSU P D, WRIGHT J, et al. Genome engineering using the CRISPR-Cas9 system [J]. Nat Protoc, 2013, 8(11): 2281-308.
[2] MEI Y, WANG Y, CHEN H, et al. Recent Progress in CRISPR/Cas9 Technology [J]. J Genet Genomics, 2016, 43(2): 63-75.
[3] LI T, YANG Y, QI H, et al. CRISPR/Cas9 therapeutics: progress and prospects [J]. Signal Transduct Target Ther, 2023, 8(1): 36.
[4] KLEINSTIVER B P, PATTANAYAK V, PREW M S, et al. High-fidelity CRISPR-Cas9 nucleases with no detectable genome-wide off-target effects [J]. Nature, 2016, 529(7587): 490-5.
[5] CHEN J S, DAGDAS Y S, KLEINSTIVER B P, et al. Enhanced proofreading governs CRISPR-Cas9 targeting accuracy [J]. Nature, 2017, 550(7676): 407-10.
[6] SLAYMAKER I M, GAO L, ZETSCHE B, et al. Rationally engineered Cas9 nucleases with improved specificity [J]. Science, 2016, 351(6268): 84-8.
[7] TAHA E A, LEE J, HOTTA A. Delivery of CRISPR-Cas tools for in vivo genome editing therapy: Trends and challenges [J]. J Control Release, 2022, 342: 345-61.
[8] ZENG Z, ZHANG X, JIANG C Q, et al. Identifying novel therapeutic targets in gastric cancer using genome-wide CRISPR-Cas9 screening [J]. Oncogene, 2022, 41(14): 2069-78.
[9] KORKMAZ G, LOPES R, UGALDE A P, et al. Functional genetic screens for enhancer elements in the human genome using CRISPR-Cas9 [J]. Nat Biotechnol, 2016, 34(2): 192-8. (in Chinese)
[10] TORRES-RUIZ R, RODRIGUEZ-PERALES S. CRISPR-Cas9 technology: applications and human disease modelling [J]. Brief Funct Genomics, 2017, 16(1): 4-12.
[11] ZHOU Y, SHARMA J, KE Q, et al. Atypical behaviour and connectivity in SHANK3-mutant macaques [J]. Nature, 2019, 570(7761): 326-31.
[12] XIE J, GE W, LI N, et al. Efficient base editing for multiple genes and loci in pigs using base editors [J]. Nat Commun, 2019, 10(1): 2852.
[13] STERNER R M, COX M J, SAKEMURA R, et al. Using CRISPR/Cas9 to Knock Out GM-CSF in CAR-T Cells [J]. J Vis Exp, 2019, (149).
[14] STADTMAUER E A, FRAIETTA J A, DAVIS M M, et al. CRISPR-engineered T cells in patients with refractory cancer [J]. Science, 2020, 367(6481).
[15] KATTI A, DIAZ B J, CARAGINE C M, et al. CRISPR in cancer biology and therapy [J]. Nat Rev Cancer, 2022, 22(5): 259-79.
[16] FRANGOUL H, ALTSHULER D, CAPPELLINI M D, et al. CRISPR-Cas9 Gene Editing for Sickle Cell Disease and beta-Thalassemia [J]. N Engl J Med, 2021, 384(3): 252-60.
[17] LEDFORD H. CRISPR treatment inserted directly into the body for first time [J]. Nature, 2020, 579(7798): 185.
Disclaimer: Shenzhen Cell Valley is committed to the research of cell and gene therapy, in order to promote emerging technologies, so that more people understand the new development of biomedicine. The content of this article is only used for information exchange, and the platform remains neutral on the content, statements and opinions of the article, and does not represent the position and views of Shenzhen Cell Valley. The relevant information in this article should not be used as a diagnosis or treatment, is not a substitute for professional medical advice, and the company's website will not assume any responsibility. The final interpretation of the content of the above statement belongs to the company's website, this statement will apply to the company's website all the time to share the article, thank you for your cooperation! Copyright description: The copyright of the article belongs to Shenzhen Cell Valley, individuals are welcome to forward to the circle of friends, media or institutions without authorization, reproduced in any form to other platforms, will be regarded as infringement. For reprinting, please contact email: contact@duanglink.com
CRISPR (Clustered Regularly Interspaced Short Palindromic Repeat), first discovered in the late 1980s, is an unusual genetic structure made up of alternating and non-repeating DNA sequences. The CRISPR/Cas system is essentially a bacterial defense mechanism against foreign DNA. Genomic analysis has shown that CRISPR and Cas proteins function as an acquired immune system and protect prokaryotic DNA from attack by phage and plasmid DNA through an RNA-guided DNA cutting system [1, 2].
CRISPR/Cas system has a variety of categories, of which CRISPR/Cas9 system is the most in-depth research, the most mature technology, the most widely used category. The CRISPR/Cas9 system consists of a single-stranded guide RNA (sgRNA) and a Cas9 protein that functions as an endonucrenase. Under the specific recognition of sgRNA, Cas9 protein reached a specific site of the genome and cut double-stranded DNA (dsDNA), resulting in double-strand break (DSB). DSBS are then repaired by cell-independent nonhomologous DNA end joining (NHEJ) or homology-directed repair (HDR). Among them, the dominant NHEJ repair is prone to mutation [3].

Figure 1. How the CRISPR/Cas9 system works (image from biorender)
二、CRISPR/Cas9 system delivery technology
The natural Cas protein still has many limitations in terms of editing efficiency and specificity, which may cut DNA double strands at misplaced loci, resulting in potential risks that significantly affect its practical application. Researchers have developed different Cas9 variants to reduce the mismatch rate and increase editing efficiency [4-6].
The delivery method of the CRISPR/Cas9 system is also one of the key factors affecting the efficiency of gene editing [7]. There are three main forms of delivery: (1) delivery of plasmid DNA encoding Cas9 and sgRNA; Delivery of Cas9 mRNA and sgRNA components; Delivery of RNP(ribonucleoprotein,Cas9 protein and sgRNA complex). The selection of different delivery modes according to different delivery forms can be divided into three main groups according to the cell entry mechanism: (1) biological methods, (2) chemical methods, and (3) physical methods. Biological methods use natural biological materials such as viral proteins, peptides or cell receptors/membranes to mediate entry into cells. This category includes viral vectors, virus-like particles (VLPs), and cell-penetrating peptides (CPPs). Chemical methods use synthetic materials, such as polymers, lipids, or metals, to facilitate entry into cells. This category includes liposomes, gold nanoparticles (AuNPs), and lipid nanoparticles (LNPs). Physical methods rely on the physical energy of electricity or ultrasound to deliver genes into cells. This category includes electroporation, sound hole effects, and microinjection.

三、CRISPR/Cas9 technology in gene and cell therapy research progress
Compared with the first and second generation gene editing technologies, CRISPR/Cas9 technology has been widely used in biomedical research due to its excellent performance in gene knockout, gene silencing, gene knockout, gene activation and epigenetic modification due to its convenient, simple and efficient operation. A report by Transparency Market Research (TMR) entitled "CRISPR and Cas Gene Market - Global Industry Analysis, Size, Share, Growth, Trends and Forecasts, 2018-2026" says that by 2026, The global CRISPR and Cas gene market is valued at $7.234.5 billion, with a CAGR of approximately 20.1% from 2018 to 2026. The use of gene editing technology for the prevention of multiple diseases, along with the increasing demand for alternative medicine for chronic diseases and increased investment by key players in Asia Pacific are expected to be the major factors driving the market from 2018 to 2026.
(1) CRISPR/Cas9 genome-wide functional screening
By constructing CRISPR-Cas9 library, some drug targets and resistance genes can be screened, analyzed and verified on a large scale, and relevant data can be provided for disease treatment. The researchers used CRISPR-Cas9 library to screen the whole genome of human gastric cancer AGS cells for potential therapeutic targets, and finally identified 41 essential genes as potential drug targets [8]. In addition, CRISPR-Cas9 library was used to screen 685 gene loci of the key transcription factor p53 and the enhancer element of ERα in cancer cells, and a total of 3 enhancer elements were identified to be related to ERα. Three enhancer elements are associated with p53 function, and only when two enhancer elements are fully combined with p53 can the cell aging process be activated [9].
(2) Model construction
Disease models are mainly used to simulate the biological and pathological characteristics of human diseases, and play a key role in the pathogenesis of diseases and drug screening.
Most animal models are constructed by knocking out specific genes [10]. Using CRISPR technology to target exon 3 of the monkey SHANK21 gene, researchers successfully constructed an ASD model of the monkey and its F1 offspring due to SHANK3 gene mutation, showing atypical autism phenotypes such as increased repetitive behaviors and social and learning deficits [11]. Point mutation knockin based on single base editing has great potential in building animal models. The researchers ended gene expression early by making single-base edits to stop codons at RAG1, RAG2, and IL2RG sites. After these embryos were implanted into surrogate pigs, it was found that piglets carrying homozygous or heterozygous mutations would suffer from severe underdevelopment of the thymus and spleen, resulting in immune deficiency and lung infection. It was also the first time that single-base editing techniques had been used in large animals [12]. In addition, the construction of cell models in vitro also helps to screen drug action targets and provide basis for revealing drug action mechanisms and disease mechanisms.
(3) Cell therapy
Immunotherapy is a method of killing tumor cells by mobilizing the patient's own immune system, which is mainly divided into immune checkpoint blocking and chimeric antigen receptor T-cell immunotherapy (CAR-T). CRISPR/Cas9 technology is different from the random integration of transgenic technology, which can accurately target target genes and carry out genetic modification. Due to the presence of endogenous HLA and TCR in allogeneic CAR-T cells, which hinder the wide application of therapy, multiple therapeutic strategies have been proposed to prepare CAR-T. Consider the important role of granulocyte-macrophage colony-stimulating factor (GM-CSF) in inducing cytokine storms. In 2019, Sterner et al. [13] used CRISPR/Cas9 to knock out the GM-CSF gene of CD19 CAR-T cells. The results of this study showed that GM-CSF secretion of CAR T cells with GM-CSF gene knockout was indeed significantly reduced, but the important function of CAR T cells was not affected. More importantly, GM-CSF-deficient CD19 CAR T cells showed significant anti-tumor effects in vivo compared with wild-type CD19 CAR T cells. In 2020, Carl June et al. [14] removed endogenous T cell receptor (TCR) and immune checkpoint molecule programmed cell death protein 1 (PD-1) to improve the function and persistence of engineered T cells. A synthetic cancer-specific TCR transgene (NY-ESO-1) was introduced to identify tumor cells (the first multiple CRISPR/Cas9 editing engineering applied to clinical trials). In the same year, the results of the first human phase I clinical trial of CRISPR-Cas9-edited PD-1 T cells in patients with advanced non-small cell lung cancer (ClinicalTrials.gov NCT02793856) were obtained by Professor Lu Youu of China, and the remaining clinical trials were shown in the following table [15].
Table 1 Ongoing clinical trials of immunotherapies designed using CRISPR technology to treat human cancer
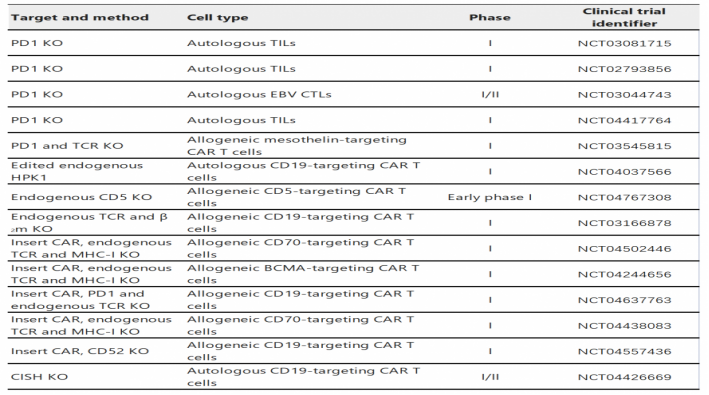
(4) Gene therapy
Gene therapy refers to the introduction of foreign normal genes into target cells to correct or compensate for diseases caused by defects and abnormal genes in order to achieve therapeutic purposes. Thanks to its high editing efficiency and the ability to simultaneously target multiple target genes, CRISPR/Cas9 technology has been used in gene therapy for diseases such as tumors, neurodegenerative diseases, hematopoietic diseases, and AIDS.
CRISPR Therapeutics has announced the early success of its treatment, called CTX001, which delivers CRISPR-Cas9 to blood stem cells via electroporation. After the patient's own hematopoietic stem cells were chemically cleared with leucoamine, the CRISPR-Cas9-edited hematopoietic stem cells were transfused back into the patient. Patients with beta-thalassemia or sickle cell disease who are treated are no longer dependent on blood transfusion therapy for a long time [16].
NTLA-2001 is being developed as a single-dose treatment for transthyroxin protein (ATTR) amyloidosis. NTLA-2001 is an in vivo gene-editing therapy designed to treat ATTR amyloidosis by reducing the concentration of TTR in the serum. The therapy is based on CRISPR/Cas, which is the first CRISPR therapy to be delivered into the bloodstream in the form of lipid nanoparticles for systemic administration [17].
Editas Medicine is developing EDIT-101, a product that is still in clinical trials. The drug uses AAV-mediated delivery of CRISPR/Cas9, targeted by sgRNA to remove the abnormal splicing donor resulting from the IVS290 mutation in the CEP26 gene, and restores normal CEP290 expression to treat congenital amaurosis. The treatment can be cured with a single dose (NCT03872479).
Reference:
[1] RAN F A, HSU P D, WRIGHT J, et al. Genome engineering using the CRISPR-Cas9 system [J]. Nat Protoc, 2013, 8(11): 2281-308.
[2] MEI Y, WANG Y, CHEN H, et al. Recent Progress in CRISPR/Cas9 Technology [J]. J Genet Genomics, 2016, 43(2): 63-75.
[3] LI T, YANG Y, QI H, et al. CRISPR/Cas9 therapeutics: progress and prospects [J]. Signal Transduct Target Ther, 2023, 8(1): 36.
[4] KLEINSTIVER B P, PATTANAYAK V, PREW M S, et al. High-fidelity CRISPR-Cas9 nucleases with no detectable genome-wide off-target effects [J]. Nature, 2016, 529(7587): 490-5.
[5] CHEN J S, DAGDAS Y S, KLEINSTIVER B P, et al. Enhanced proofreading governs CRISPR-Cas9 targeting accuracy [J]. Nature, 2017, 550(7676): 407-10.
[6] SLAYMAKER I M, GAO L, ZETSCHE B, et al. Rationally engineered Cas9 nucleases with improved specificity [J]. Science, 2016, 351(6268): 84-8.
[7] TAHA E A, LEE J, HOTTA A. Delivery of CRISPR-Cas tools for in vivo genome editing therapy: Trends and challenges [J]. J Control Release, 2022, 342: 345-61.
[8] ZENG Z, ZHANG X, JIANG C Q, et al. Identifying novel therapeutic targets in gastric cancer using genome-wide CRISPR-Cas9 screening [J]. Oncogene, 2022, 41(14): 2069-78.
[9] KORKMAZ G, LOPES R, UGALDE A P, et al. Functional genetic screens for enhancer elements in the human genome using CRISPR-Cas9 [J]. Nat Biotechnol, 2016, 34(2): 192-8. (in Chinese)
[10] TORRES-RUIZ R, RODRIGUEZ-PERALES S. CRISPR-Cas9 technology: applications and human disease modelling [J]. Brief Funct Genomics, 2017, 16(1): 4-12.
[11] ZHOU Y, SHARMA J, KE Q, et al. Atypical behaviour and connectivity in SHANK3-mutant macaques [J]. Nature, 2019, 570(7761): 326-31.
[12] XIE J, GE W, LI N, et al. Efficient base editing for multiple genes and loci in pigs using base editors [J]. Nat Commun, 2019, 10(1): 2852.
[13] STERNER R M, COX M J, SAKEMURA R, et al. Using CRISPR/Cas9 to Knock Out GM-CSF in CAR-T Cells [J]. J Vis Exp, 2019, (149).
[14] STADTMAUER E A, FRAIETTA J A, DAVIS M M, et al. CRISPR-engineered T cells in patients with refractory cancer [J]. Science, 2020, 367(6481).
[15] KATTI A, DIAZ B J, CARAGINE C M, et al. CRISPR in cancer biology and therapy [J]. Nat Rev Cancer, 2022, 22(5): 259-79.
[16] FRANGOUL H, ALTSHULER D, CAPPELLINI M D, et al. CRISPR-Cas9 Gene Editing for Sickle Cell Disease and beta-Thalassemia [J]. N Engl J Med, 2021, 384(3): 252-60.
[17] LEDFORD H. CRISPR treatment inserted directly into the body for first time [J]. Nature, 2020, 579(7798): 185.
Disclaimer: Shenzhen Cell Valley is committed to the research of cell and gene therapy, in order to promote emerging technologies, so that more people understand the new development of biomedicine. The content of this article is only used for information exchange, and the platform remains neutral on the content, statements and opinions of the article, and does not represent the position and views of Shenzhen Cell Valley. The relevant information in this article should not be used as a diagnosis or treatment, is not a substitute for professional medical advice, and the company's website will not assume any responsibility. The final interpretation of the content of the above statement belongs to the company's website, this statement will apply to the company's website all the time to share the article, thank you for your cooperation! Copyright description: The copyright of the article belongs to Shenzhen Cell Valley, individuals are welcome to forward to the circle of friends, media or institutions without authorization, reproduced in any form to other platforms, will be regarded as infringement. For reprinting, please contact email: contact@duanglink.com